amd
AMD: Counteracting Conversion
A look at the literature on the risk-decreasing techniques
Andrew S. Gurwood, O.D., F.A.A.O., DIPL., Julie K. Hutchinson, O.D., F.A.A.O. and Marc D. Myers, O.D., F.A.A.O., Philadelphia
In general, the risk to dry AMD patients progressing to wet AMD for any given five-year period is approximately 14% to 20%.1,2 (See “AMD Background,” at the end.) In addition, the risk through five-years for developing wet AMD in the fellow eye is 10% for those whose fellow eye has neither large drusen or pigment clumps (hyperpigmentation of the retinal pigment epithelium [RPE]), 30% for fellow eyes containing either large drusen or pigment clumps of the RPE and 50% for fellow eyes that have both pigment clumps of the RPE and large drusen. Various management techniques exist which permit an attempt to protect dry AMD patients from converting to wet AMD (choroidal neovascularization [CNV]).
Diet
Several studies demonstrate that a healthy diet can decrease one's risk of progressing to wet AMD. For example, one study revealed that higher fish consumption and nuts reduced the risk of progression.3,4 Meanwhile, higher total fat intake, higher vegetable fat intake, saturated, monounsaturated, polyunsaturated and transunsaturated fat intake all increased the likelihood of progression. Further, animal fat intake was linked with a two-fold increased risk of progression.3
Supplementation
With an absorption spectrum between 400nm to 540nm, research shows that retinal carotenoids (lutein, zeaxanthin, mesozeaxathin), which comprise the dense macular pigment, are integral in blocking harmful blue ultraviolet light. This light provokes the physiology that results in retinal damage.5 Additionally, the literature reveals that carotenoids serve as antioxidants and have been implicated in preventing degenerative eye disease via free radical scavenging and the reduction of reactive oxygen species.5
The Age-related Eye Disease Study (AREDS), involving more than 3,600 patients, revealed that the prospective administration of purified vitamin C, vitamin E, beta-carotene, zinc and copper (ascorbic acid, 500 mg/d; vitamin E, 400 IU/d; beta-carotene, 15 mg/d, minerals zinc oxide, 80 mg/d; and cupric oxide, 2 mg/d) (e.g. the “AREDS formulation”) reduced the risk of developing advanced AMD from 28% to 20% and the rate of at least moderate vision loss from 29% to 23%.2,6 AREDS researchers have applied their findings to an estimated eight million U.S. persons at least age 55, at risk for developing advanced AMD in whom the AREDS formulation should be considered. Of these people, it's projected that 1.3 million would develop advanced AMD if no treatment were given to reduce the risk. If all those at risk received the appropriate studied supplement formulation, more than 300,000 of them could potentially avoid conversion to advanced AMD reducing the risk of vision loss for the given five-year period.6
In addition, AREDS researchers completed work comparing individuals ingesting high doses of antioxidants or zinc with mortality.7 Participants in the study were randomly assigned to receive oral supplements of high-dose antioxidants, zinc, antioxidants plus zinc or placebo. The result: Those randomly assigned to receive zinc had lower mortality than those not taking zinc.7
As a result of the AREDS findings, several companies have since released “AREDS formulations,” which have been shown as a useful tool for slowing AMD progression.7-12 Given the hypothesis that beta-carotene levels are inversely related to AMD and amidst increasing evidence that visible and ultraviolet light can damage the retina through superoxide radical production, the National Eye Institute is currently sponsoring The Randomized Trial of Beta–Carotene and Macular Degeneration study.13 The study's goal: to determine whether one 50mg beta-carotene capsule taken on alternate days protects against AMD development and whether additional risk factors emerge after controlling for other potential confounding factors.13
A caveat: Studies have revealed increases in lung cancer among smokers who use beta-carotene.13-17 In addition, recent survey data as well as laboratory animal studies continue to show an inverse association between beta-carotene and cancer risk in non-smoking subjects.17 Because beta-carotene is the major vitamin A source for the majority of the world's population, it's critical to define the safe levels of intake from foods and supplements for all.14 The unexpected findings of increased lung cancer in beta-carotene supplemented smokers, seen in the Alpha-Tocopherol Beta-Carotene study (ATBC) and the Beta-Carotene and Retinol Efficacy Trial (CARET), have resulted in the need for research to define beta-carotene's mechanism(s) of action.13-17
The Lutein Antioxidant Supplementation Trial (LAST) revealed that all patients who took lutein had a mean eye macular pigment optical density (MPOD) increase of approximately 0.09 log units from baseline.18 (Research has shown a high MPOD may decrease a patient's risk for AMD-related vision loss.19) Also, Snellen visual acuity improved 5.4 letters in those taking 10mg of lutein and 3.5 letters in those taking lutein and antioxidants. In addition, contrast sensitivity improved in both groups. Further, a net subjective improvement in the Amsler grid measurement was seen in the lutein group.18,19 Patients who received placebo had no significant changes in any of the measured findings.
The AREDS 2 study, currently underway, seeks to answer the question: “Is the current AREDS formulation sufficient, or should omega-3 fatty acids (found in fish and nuts, among other foods) and/or lutein with zeaxan thin be added?”20-22 A secondary endpoint was designed to determine whether the zinc levels in the original formulation were excessive and whether beta-carotene can be removed altogether, making one version of the supplement appropriate for everyone.20-22
Finally, a study revealed that dry AMD patients older than age 60 were able to decrease their CNV formation rate via statin drugs or aspirin.23-28 While statin drugs are not routinely prescribed in a protective role, this information supports the use of a protective aspirin therapy.
Optical coherence tomography
Optical coherence tomography continues to enable clinicians to observe the retinal and subretinal histological strata in detail via 3D cross-sectional images. As a result, these devices enable the mapping of drusen, subretinal fluid, retinal pigment epithelium (RPE) detachment and CNV, making them ideal for AMD detection and monitoring.29-31 Spectral domain OCT devices—the latest technology—permit the calculation of volume of lesions detected, enhancing the clinician's ability to closely monitor progression.30 Changes in volume up or down can signify lesion growth or shrinkage.
Fundus autofluorescence imaging
Fundus autofluorescence imaging, or FAF, permits the non-invasive visualization of lipofuscin accumulation.29,30,32,33 Lipofuscin is a polyunsaturated fat that remains in overabundance secondary to errant RPE elimination and is a primary constituent of drusen formation.33 The RPE, which phagocytizes the outer segments of photoreceptors, accumulates autofluorescent lipofuscin.33 Loss of RPE autofluorescence signifies histological disruption or death of the RPE and photoreceptor layers and could represent early disease or a marker of susceptibility.
Visual field testing
The Amsler grid may monitor the stability of suspicious or involved maculae.9,20,34 Specifically, this uniform arrangement of small squares, allows one, in theory, to detect changes in their visual functioning, such as scotoma or metamorphopsia formation. The test permits the patient to monitor their visual status at home, though it's a low sensitivity screening device. Cortical fill-in, poor fixation and the crowding phenomenon create observer issues that can garner false negative results.34,35 Something else to consider: Amsler grids can suffer from poor patient understanding and compliance and fall victim to the subjective inattention of patients who are traditionally not well-trained observers. For this reason, regularly scheduled follow-up exams with objective tests capable of quantification are necessary.
Vernier, or “hyperacuity,” (the ability to align two lined segments) is being utilized to enable the early identification of RPE elevations that indicate intermediate dry-to-wet AMD conversion. The histological basis of “hyperacuity” is the regular arrangement of photoreceptor cells in the retina. A spatial shift of the RPE or the neurosensory retina disorganizes the cells, causing objects to be perceived in a false location.34,36 Two vernier acuity devices are currently available: the Foresee Preferential Hyperacuity Perimeter (PHP) (Reichert Technologies, Depew, NY) and the PreView PHP (Carl Zeiss Meditec, Dublin, Calif.) Both measure the central 14° of field, and their ability to detect misalignments falls in the magnitude of three-to-six seconds of arc. Also, both devices are 82% accurate for detecting new CNV. A recent study on PHP's efficacy and usability for monitoring high-risk intermediate AMD patients revealed it maintained a low false-positive rate (8.1%).37
In July, the FDA cleared the MAIA fundus perimeter (Center-Vue, Italy) for marketing. It measures macular sensitivity (suprathreshold and threshold algorithms available), fixation stability and fixation locus, also enabling the early detection of retinal disease, including AMD.38 Further, the device contains a scanning laser ophthalmoscope for retinal imaging.
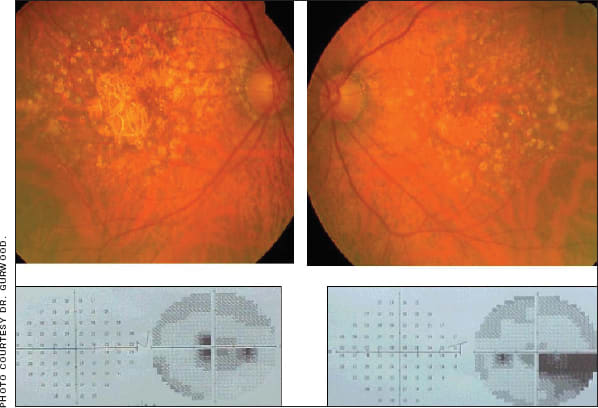
This patient developed dry AMD OU with corresponding visual field loss.
Heterochromic flicker photometry
Heterochromic flicker photometry (HFP) uses dual light stimuli of short and long wavelength via spectral absorption to approximate MPOD in vivo at specific parafoveal locations.5 The MPOD measurement is then calculated by taking the logarithm of the luminance ratios of the presented stimuli. Currently two HFP devices are available: MacuScope (Marco, Jacksonville, Fla.) and QuantifEye (Zeavision, Chesterfield, MO). The information gleaned from these tests must be batched with other data to ensure a clear trend.
New instruments are permitting both timely diagnosis and enhanced accuracy. Also, evidence-based support of oral supplements have made the prevention of the conversion of dry-to-wet AMD possible. These user-friendly adjuncts can postpone pathological changes in the population at risk and augment protection from the genesis of the pathology in the healthy. OM
AMD Background |
---|
AMD, the deterioration of the macular photore, is the leading cause of acquired legal blindness in the United States for white persons older than age 65, and it's bilateral in 55% of cases.1,6,7-9,10-12,39-47 In the United States alone, a total of 1.75 million individuals older than age 40 suffer from some form of the disease with another seven million having drusen (focal thickenings of Bruch's membrane - vitronectin, apolipoproteins B and E, complement, lipid)—a potential precursor to AMD.47 The disease is present in approximately 10% to 18% of the American population older than age 52 and in up to 30% to 33% of Americans older than age 75.3,4,8,10 It is estimated that by 2020, some 2.95 million Americans will suffer from AMD's visually impairing sequellae—an increase that could approach 50%.47 The risk factors for the disease have the common side effect of reducing retinal oxygenation while increasing reactive oxygen species and unstable free radicals. These risk factors include obesity, cardiovascular disease, smoking, advanced age, white race, AMD family history (genetics), previous lung infection history in those who have a cardiovascular history, hyperopia, exposure to ultraviolet light and decreased grip strength.1,2,8,48 All AMD forms possess initial common changes within the macular RPE. Some researchers postulate these changes are initiated by isolated interruptions of homeostasis within RPE regions, Bruch's membrane and/or the choriocapillaris that subserve the macular region. These interruptions are thought to result in eventual photoreceptor degradation. Through time, the accumulation of undigested extracellular debris alters the Bruch's membrane composition (i.e., increased lipid and protein content) and permeability (e.g., decreased permeability to water-soluble constituents in plasma, amino acid transport and possibly decreased removal of extruded RPE-derived cytoplasmic debris across the Bruch's membrane).2,30,32,33 It is thought that these changes complicate the diffusion of waste products, hormones and nutrients, including oxygen and vitamin A, to and from the RPE. In response to this metabolic distress, the RPE itself may produce substances that stimulate choroidal neovascular membrane (CNV) growth. All the while, the photoreceptors disintegrate, causing the inner nuclear layer of the macular photoreceptors to collapse and initialize outer retinal layer death.6,7 The earliest clinical manifestation of AMD are drusen. Most of the molecular constituents of drusen are synthesized as a consequence of a dysfunctional RPE, neural retina or choroidal cells, however, some are derived from extraocular sources, which may reflect the association of some atherosclerotic and cardiovascular risk factors.2,43 Wet AMD results when the macular RPE/Bruch's barrier is compromised by new, weak and leaky blood vessels. This new vessel growth is stimulated, at least in part by angiogenic factors released within the damaged retinal pigment epithelial tissues secondary to the pathologic process.2,49 The vessels grow into the retina from the choriocapillaris to form CNV membranes termed occult when they are poorly defined or classic when easily defined. The incompetent vasculature of these membranes may leak serosanguinous fluid at the level or adjacent to the level at which they reside, causing RPE detachment, sensory retinal detachment, subretinal or intraretinal bleeding and fibrovascular, disciform scarring.2,8,41,49 The clinical signs of dry AMD are comprised of drusen of the posterior pole, granular clumping and disorganization of the RPE in the macular area, macular RPE hyperplasia and degeneration of the outer retinal layers with circumscribed areas of geographic atrophy of the RPE.8,4,9 The clinical signs associated with wet AMD: hard and soft drusen, subretinal thickening secondary to classic or occult CNV, which produces a grayish-green subretinal hue upon observation, subretinal, intraretinal or vitreous hemorrhage, subretinal and intraretinal exudation with serosanguinous fluid accumulation and fibrovascular scar formation (disciform scarring).2,7 ![]() This patient developed wet AMD with disciform scarring OS. |
References
1. Deglin EA. Pigment Epithelial/Bruch's Membrane/Choroidal Diseases-Part I. In: Diseases of The Retina and Vitreous. Philadelphia, PA:PCO press 1995:1-32.
2. Zarbin MA. Current concepts in the pathogenesis of age-related macular degeneration. Arch Ophthalmol. 2004 Apr;122(4):598-614.
3. Seddon JM, Cote J, Rosner B. Progression of age-related macular degeneration: association with dietary fat, transunsaturated fat, nuts, and fish intake. Arch Ophthalmol. 2003 Dec;121(12):728-37.
4. Seddon JM, Rosner B, Sperduto RD, et al. Dietary fat and risk for advanced age-related macular degeneration. Arch Ophthalmol 2001;119(8):1191-9.
5. Howells O, Eperjesi F, Bartlett H. Measuring macular pigment optical density in vivo: a review of techniques. Graefes Arch Clin Exp Ophthalmol. 2011. Mar;249(3):315-47.
6. Bressler NM, Bressler SB, Congdon NG, et al. Age-Related Eye Disease Study Research Group. Potential public health impact of Age-Related Eye Disease Study results: AREDS report No. 11. Arch Ophthalmol. 2003 Nov;121(11):1621-4.
7. Clemons TE, Kurinij N, Sperduto RD; AREDS Research Group. Associations of mortality with ocular disorders and an intervention of high-dose antioxidants and zinc in the Age-Related Eye Disease Study: AREDS Report No 13. Arch Ophthalmol. 2004;122(5):716-26.
8. Gurwood, AS, Gerson J. Wet AMD: New Theories and New Treatments: Part I. Optometric Management 2008 March;43(3):53-67.
9. Klein ML. Macula: Age-Related Macular Degeneration. In : Fraunfelder FT, Roy F.H. Current Ocular Therapy 3. Philadelphia, PA; WB Saunders Co 1990:623-627.
10. Alexander LJ. Ocular Vitamin Therapy. A review and assessment. Optom Clin. 1992;2(1):1-34.
11. Mitchell P, Smith W, Cumming RG, et al. Nutritional factors in the development of age-related eye disease. Asia Pac J Clin Nutr. 2003;12Suppl:S5.
12. Hammond BR Jr, Johnson MA. The age-related eye disease study (AREDS). Nutr Rev. 2002 Sep;60(9):283-8.
13. Christen WG, Manson JE, Glynn RJ, et al. Beta-carotene supplementation and age-related maculopathy in a randomized trial of US physicians. Arch Ophthalmol. 2007 March;125(3):333-9.
14. Goodman GE. Prevention of lung cancer. Curr Opin Oncol. 1998 March;10(2):122-6. Review.
15. Albanes D, Heinonen OP, Taylor PR, et al. Alpha-Tocopherol and beta-carotene supplements and lung cancer incidence in the alpha-tocopherol, beta-carotene cancer prevention study: effects of base-line characteristics and study compliance. J Natl Cancer Inst. 1996 Nov 6;88(21):1560-70.
16. Omenn GS, Goodman GE, Thornquist MD, et al. Risk factors for lung cancer and for intervention effects in CARET, the Beta-Carotene and Retinol Efficacy Trial. J Natl Cancer Inst. 1996 Nov 6;88(21):1550-9.
17. Bendich A. From 1989 to 2001: What have we learned about the "biological actions of beta-carotene?" J Nutr. 2004 Jan;134(1):225S-230S.
18. Richer S, Stiles W, Statkute L, et al. Double-masked, placebo-controlled, randomized trial of lutein and antioxidant supplementation in the intervention of atrophic age-related macular degeneration: the Veterans LAST study (Lutein Antioxidant Supplementation Trial). Optometry. 2004; Apr;75(4):216-30.
19. Richer S, Devenport J, Lang, JC. LAST II: Differential temporal responses of macular pigment optical density in patients with atrophic age-related macular degeneration to dietary supplementation with xanthophylls. Optometry. 2007 May;78(5):213-9.
20. Loewenstein A. Malach R, Goldstein M, et al. Replacing the Amsler grid: a new method for monitoring patients with age-related macular degeneration. Ophthalmology 2003 May;110(5):966-70.
21. Seddon JM, George S, Rosner B. Cigarette smoking, fish consumption, omega-3 fatty acid intake, and associations with age-related macular degeneration: the US Twin Study of Age-Related Macular Degeneration. Arch Ophthalmol. 2006; Jul;124(7):995-1001
22. Hodge WG, Schacter HM, Barnes D, et al. Efficacy of omega-3 fatty acids in preventing AMD: a systematic review. Ophthalmology. 2006; Jul;113(7):1165-72; quiz 1172-3, 1178.
23. Alves-Rodrigues A, Shao A. The science behind lutein. Toxicol Lett 2004; Apr 15;150(1):57-83.
24. Mitchell P, Smith W, Cumming RG, et al. Nutritional factors in the development of age-related eye disease. Asia Pac J Clin Nutr 2003;12 Suppl:S5.
25. Seddon JM, Cote J, Rosner B. Progression of age-related macular degeneration: association with dietary fat, transunsaturated fat, nuts, and fish intake. Arch Ophthalmol 2003; Dec;121(12):1728-37.
26. Seddon JM, Rosner B, Sperduto RD, et al. Dietary fat and risk for advanced age-related macular degeneration. Arch Ophthalmol 2001; Aug;119(8):1191-9.
27. Wilson HL, Schwartz DM, Bhatt HR, et al. Statin and aspirin therapy are associated with decreased rates of choroidal neovascularization among patients with age-related macular degeneration. Am J Ophthalmol 2004 Apr;137(4):615-24.
28. Age-Related Eye Disease Study Research Group. Risk factors associated with age-related macular degeneration. A case-control study in the age-related eye disease study: age-related eye disease study report number 3. Ophthalmology 2000;Dec;107(12):2224-32.
29. Schmitz-Valckenberg S, Fleckenstein M, Göbel AP, et al. Optical coherence tomography and autofluorescence findings in areas with geographic atrophy due to age-related macular degeneration. Invest Ophthalmol Vis Sci. Jan 5;52(1):1-6. Print 2011 Jan.
30. Gregori G, Wang F, Rosenfeld PJ, et al. Spectral domain optical coherence tomography imaging of drusen in nonexudative age-related macular degeneration. Ophthalmology. 2011 Mar 7. [Epub ahead of print]
31. Schmitz-Valckenberg S, Fleckenstein M, Scholl HP, Holz FG. Fundus autofluorescence and progression of age-related macular degeneration. Surv Ophthalmol. 2009 Jan-Feb;54(1):96-117.
32. Delori FC, Dorey CK, Staurenghi G, et al. In vivo florescence of the ocular fundus exhibits retinal pigment epithelium lipofuscin characteristics. Invest Ophthalmol Vis Sci. 1995 Mar;36(3):718-29.
33. Choudhry N, Giani A, Miller JW. Fundus autofluorescence in geographic atrophy: a review. Semin Ophthalmol. 2010 Sep-Nov;25(5-6):206-13.
34. Loewenstein A, Ferencz JR, Lang Y, et al. Toward earlier detection of choroidal neovascularization secondary to age-related macular degeneration: multicenter evaluation of a preferential hyperacuity perimeter designed as a home device. Retina. 2010 Jul-Aug;30(7):1058-64.
35. Loewenstein A. The significance of early detection of age-related macular degeneration: Richard & Hinda Rosenthal Foundation Lecture, The Macula Society 29th Annual Meeting. Retina. 2007;Sep;27(7):873-8.
36. Goldstein M, Loewenstein A, Barak A, et al. Results of a multicenter clinical trial to evaluate the preferential hyperacuity perimeter for detection of age-related macular degeneration. Retina. 2005 Apr-May;25(3):296-303.
37. Stur M, Manor Y. Long-term monitoring of age-related macular degeneration with preferential hyperacuity perimetry. Ophthalmic Surg. Lasers Imaging. 2010 Nov 1;41(6):598-606.
38. MAIA Macular Integrity Assessment website. www.ellex.com/assets/files/products/maia/MAIA_Overview.pdf. Accessed March 21 2011.
39. Alexander LJ. Exudative and Nonexudative Macular Disorders In: Alexander, L.J. Primary Care of The Posterior Segment. East Norwalk, CT: Appleton and Lange 1994:277-344.
40. Macular Photocoagulation Study Group. Argon laser photocoagulation for senile macular degeneration: Results of a randomized clinical trial. Arch of Ophthalmol. 1982;100(4):912-918.
41. Melberg NS, Thomas MA, Burgess DB. The surgical removal of subfoveal choroidal neovascularization: Ingrowth site as a predictor of visual outcome. Retina 1996;16(3):190-5.
42. Smiddy WE, Fine SL. Prognosis of patients with bilateral drusen. Ophthalmology 1984 Mar;91(3):271-7.
43. Seddon JM, Gensler G, Milton RC, et al. Association between C-reactive protein and age-related macular degeneration. JAMA. 2004 Feb 11;291(6):704-10.
44. Yannuzzi LA, Ciardella A, Spaide RF, et al. The expanding clinical spectrum of idiopathic polypoidal choroidal vasculopathy. Arch Ophthalmol. 1997 Apr;115(4):478-85.
45. Lafaut BA, Leys AM, Snyers B, et al. Polypoidal choroidal vasculopathy in Caucasians. Graefes Arch Clin Exp Ophthalmol. 2000 Sep;238(9):752-9.
46. Ciardella AP, Donsoff IM, Huang SJ, et al. Polypoidal choroidal vasculopathy. Surv Ophthalmol. 2004 Jan-Feb;49(1):25-37.
47. Congdon N, O'Colmain B, Klaver CC, et al. Causes and prevalence of visual impairment among adults in the United States. Arch Ophthalmol. 2004 Apr;122(4):477-85.
48. Nolan JM, Kenny R, O'Regan C, et al. Macular pigment optical density in an ageing Irish population: The Irish Longitudinal Study on Ageing. Ophthalmic Res. 2010;44(2):131-9.
49. Skeie JM, Zeng S, Faidley EA, et al. Angiogenin in age-related macular degeneration. Mol vis. 2011 Feb 23;17:576-82.
Dr. Gurwood is a professor at PCO at Salus University in Elkins Park, Pa. Dr. Myers works at the Coatesville VA Medical Center, in Coatesville, Pa., and Dr. Hutchinson practices privately in Webster Groves, Mo. Send comments to optometricmanagement@gmail.com. |